RESEARCH FOCUS
The Paulino Lab is focused on elucidating the mechanism of action of membrane transporters (secondary- and primary-active) and channels on a molecular level. Although of high pharmacological relevance (> 60% of current drugs target membrane proteins), how their three-dimensional structure relates to their function and vice-versa is poorly understood and requires an interdisciplinary approach at the interface of biology, chemistry and physics. To address these questions, we employ cryo-electron microscopy and contrast our findings with comprehensive functional studies. Projects in our group are driven by the fundamental question of how membrane transporters work: how does the protein architecture translate into function; what is the exact mechanism of action during translocation of compounds from one side of the membrane to the other; how are they regulated; how is malfunction related to a disease? Over the past years, we have gained a particular interest in membrane transport proteins that fall out-of-the-box, challenging the conceptual boundaries present when classifying transport mechanisms into merely primary-active transporters, secondary-active transporters, or channels. It is becoming increasingly evident that in the course of evolution conserved protein architectures not only evolved from one another but can merge together to adapt to different environmental and cellular requirements.
Research topics
SLC9C1
Think like a channel, act like a transporter;
The newly characterized sperm-specific Na+/H+ exchanger SLC9C1 stands out by its unique tripartite domain composition. It unites a classical solute carrier unit with regulatory domains usually found in ion channels, namely, a voltage-sensing domain and a cyclic-nucleotide binding domain, which makes it a mechanistic chimera and the first known secondary-active transporter activated strictly by membrane voltage. SLC9C1 is a remarkable chimeric protein demonstrating the adaptable repertoire of nature’s building blocks and mechanistic concepts. In general terms, SpSLC9C1 architecture could be described as ‘molecular lego’, where one protein combines independently evolved functional units to accommodate to different environmental and cellular needs. It thereby links different signaling pathways in sperm (voltage-activation, pHi signaling and cAMP signaling). Our structures of the sea urchin SpSLC9C1 in the absence and presence of ligands reveal the overall domain arrangement and new structural coupling elements. They allow us to propose a gating model, where movements in the voltage sensor indirectly cause the release of the exchanging unit from a locked state through long-distance allosteric effects transmitted by the newly characterized coupling helices. We further propose that modulation by its ligand cyclic AMP occurs by means of disruption of the cytosolic dimer interface, which lowers the energy barrier for S4 movements in the voltage-sensing domain. As SLC9C1 members have been shown to be essential for male fertility, including in mammals, our structure represents a potential new platform for the development of new on-demand contraceptives.
see:
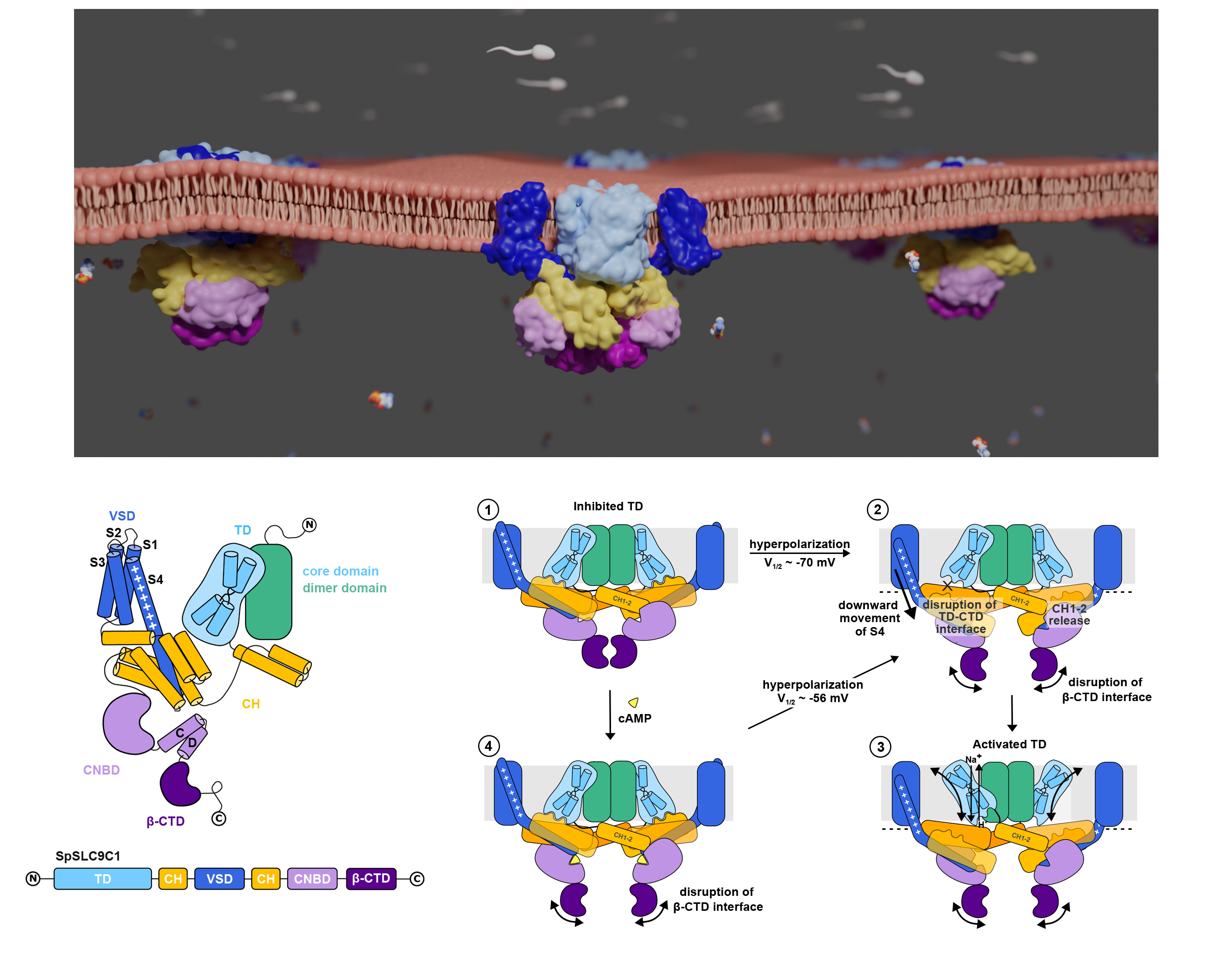
__________
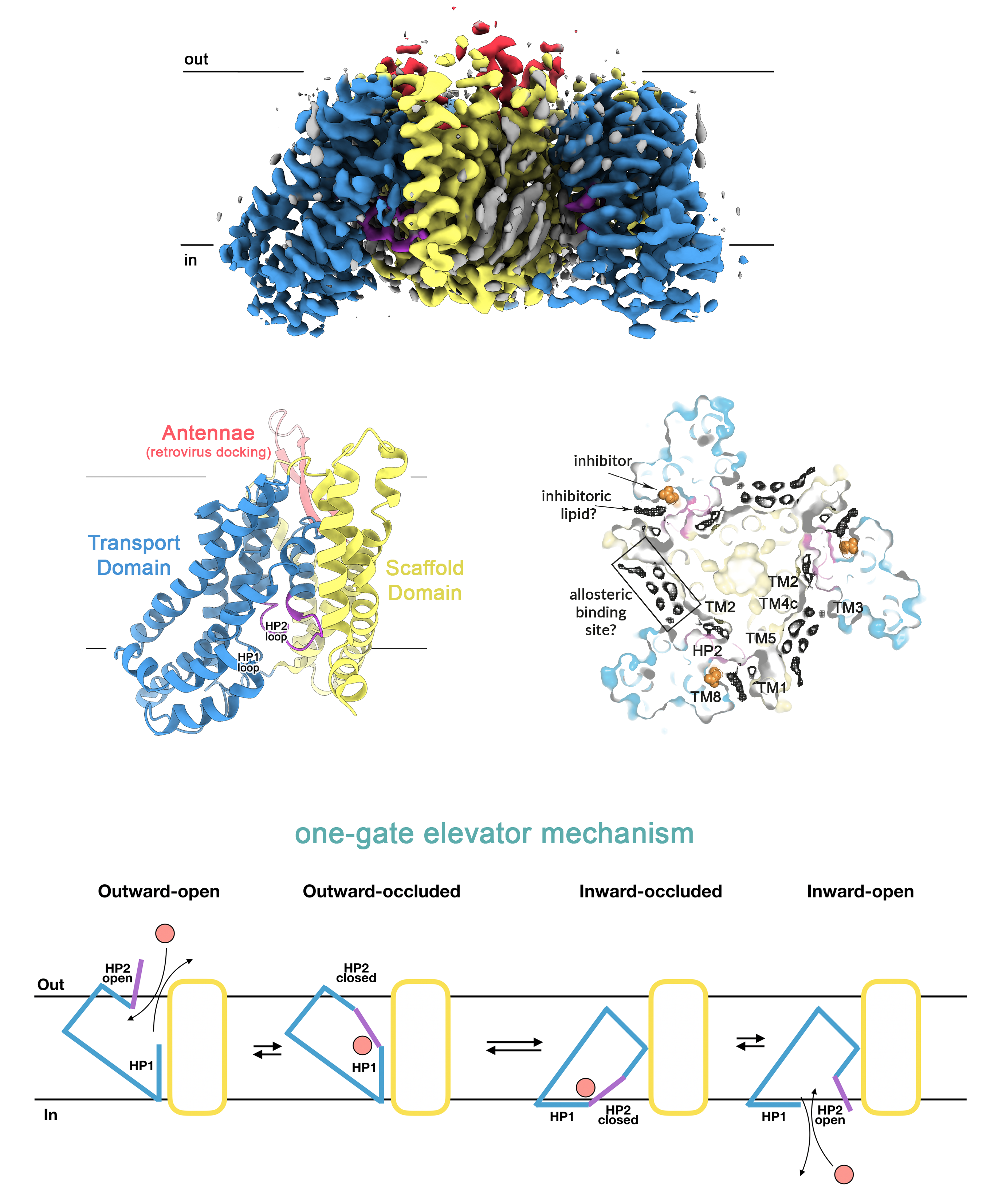
ASCT2
Anticancer drug target with an elevator mechanism
Other projects have, apart from a mechanistic question, also a social-economical application. This is demonstrated in our studies of the human neutral amino-acid transporter ASCT2 from the SLCA1 family that also comprises the human excitatory amino acid transporters EAAT1-5 and the prokaryotic GltPhand GltTk. ASCT2 is the main source of glutamine uptake in human cells, which is strongly linked to cancer cell growth, poor patient survival and a new hot-target in cancer therapy. We were able to solve the first structures of the human ASCT2 in a substrate-bound inward-occluded state and more recently a substrate-free inward-open state of ASCT2. The latter represents the first structure of any SLCA1 in an inward-open state, and answers a long-lasting key mechanistic question. It was known that these transporters work like an elevator, in which the substrate is translocated across the cell membrane by a large displacement of the transport domain, whereas a small movement of hairpin 2 (HP2) gates the extracellular access to the substrate-binding site. However, it has remained unclear how substrate binding and release is gated on the cytoplasmic side. Strikingly, our data show that the same structural element (HP2) serves as a gate in the inward-facing as in the outward-facing state, revealing that SLC1A transporters work as one-gate elevators instead of two-gate elevators as previously assumed. This observation is of great fundamental interest, but also has potential implications for drug design. A prominent consequences of the one-gate elevator mechanism is that large protein movements take place in the cell membrane during transport. We were able to identify several unassigned densities near the gate and surrounding the scaffold domain, which may represent potential allosteric binding sites and guide the design of lipidic-inhibitors for anticancer therapy. This work is done in collaboration with the group of Dirk Slotboom, one of the pioneers in SLCA1, and the bioinformatics and medicinal chemistry labs of Avner Schlessinger and Christof Grevwer.
see:
__________
OpuA
Osmoregulatory ABC transporter
see:

__________
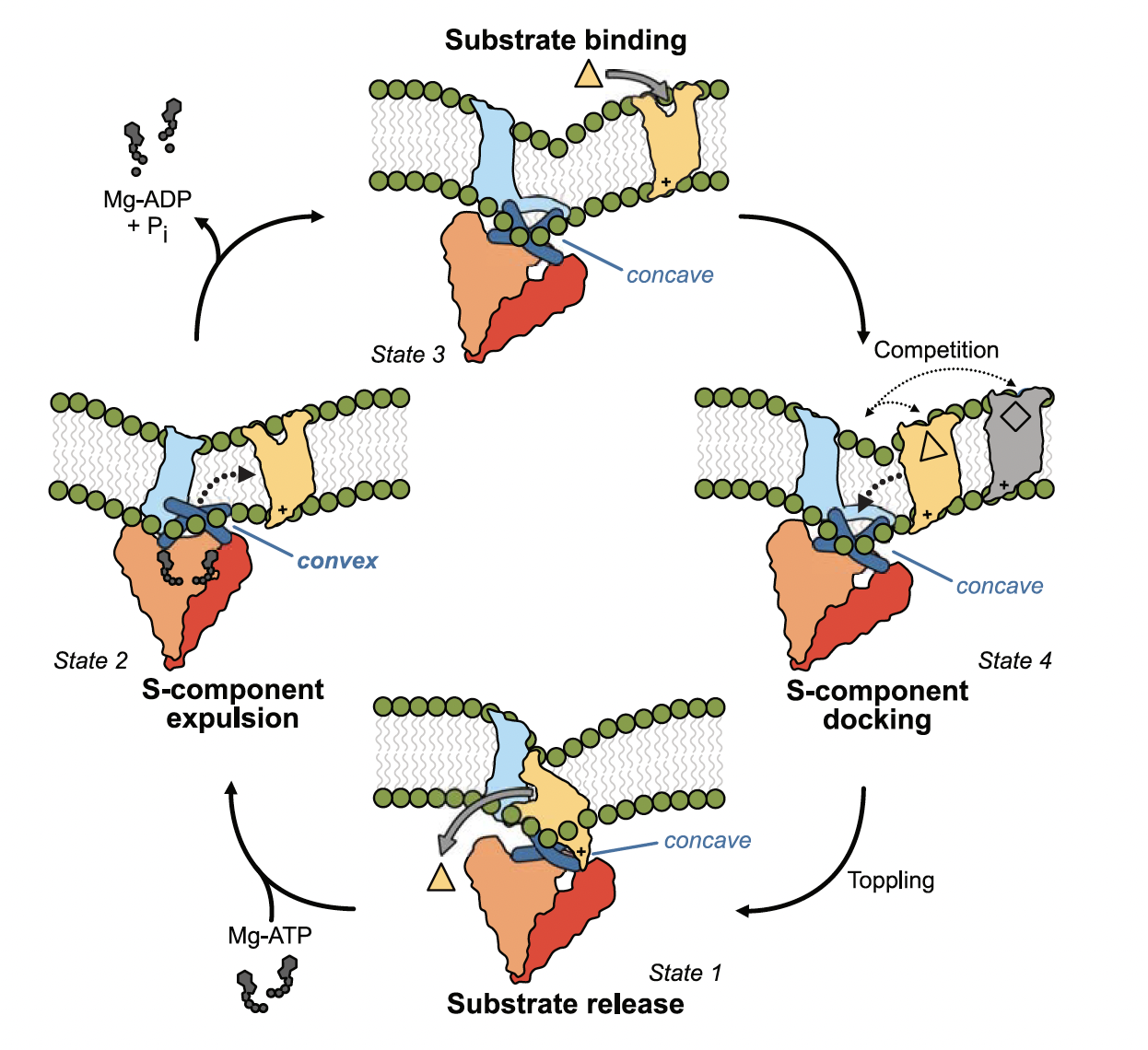
ECF
Bilayer-mediated toppling mechanism
Gram-positive bacteria that lack biosynthesis pathways for micronutrients such as vitamins use a class of membrane proteins to acquire them from the environment. These membrane proteins are called energy-coupling factor (ECF) transporters and function in an unusual way. They belong to the phylogenetically and mechanistically distinct type III clade of the ATP-binding cassette (ABC) transporter superfamily, which was first described in the late 1970, with the molecular identity only uncovered in the late 2000s. Here, a tripartite ATP-hydrolysing motor (ECF module), composed of a membrane-embedded scaffold protein (EcfT) and two cytosolic nucleotide-binding proteins or ATPase subunits (often hetero-dimeric – EcfA and EcfA’), forms a functional core complex with a membrane-embedded substrate-binding and -translocating protein (S-component). The latter rotates within the membrane to bring a molecule from the outside to the inside of the cell. But how can this motion occur within a bilayer environment and which effect has the lipid composition? We studied this enigmatic expulsion step by cryo-EM and reveal that ATP induces a concave-to-convex shape change of two long helices in the motor, thereby destroying the S-component’s docking site and allowing for its dissociation. We show that adaptation of the surrounding lipid bilayer to the conformational state of the motor may favour expulsion of the substrate-free S-component when ATP is bound and docking of the substrate-loaded S-component after hydrolysis. Our work provides a picture of bilayer-assisted chemo-mechanical coupling in the transport cycle of ECF transporters.
Notably, this project also served as a proof of principle to demonstrate the power of cryo-EM studies of membrane proteins at lower voltage electron microscopes, often downgraded as ‘only’ screening machines. Here, we were able to obtain a 2.6 Å resolution map of this 115 kDa asymmetric protein complex at 200 keV.
This work is done in collaboration with the group of Dirk Slotboom.
see:
__________
KdpFABC
A true chimera: a P-type ATPase hijacks a channel
P-type ATPases ubiquitously pump cations across biological membranes to maintain vital ion gradients. Among those, the chimeric K+ uptake system KdpFABC found only in a few prokaryotes is unique. This 157kDa complex is expressed under stress conditions, when the external K+ concentration is too low for ubiquitous K+-transporters to maintain the internal potassium concentration. KdpFABC is composed of four subunits, whereby the KdpA subunit resembles a K+-channel and the KdpB subunit is classified as a P-type ATPase (primary-active transporter). While ATP hydrolysis is accomplished by the subunit KdpB, K+ has been initially assumed to be solely transported via KdpA. By contrast, our structures of KdpFABC led us to propose a so far unprecedented transport mechanism via an intersubunit tunnel, where K+ is translocated through both KdpA and KdpB subunits. It units the alternating-access mechanism of actively pumping P-type ATPases with the high affinity and selectivity of K+-channels. This way, KdpFABC functions as a true chimeric complex, synergizing the best features of otherwise separately evolved transport mechanisms. Further, we gained insights into the coupling mechanism, where a phenylalanine is directly linked to ion propagation and turnover by: (1) acting as a gatekeeper to hinder unspecific access to the proximal and canonical binding site from the intersubunit tunnel, preventing an uncoupling of ATP hydrolysis from K+; (2) being involved in ion coordination and progression via its π-electron system; and (3) regulating the rate-limiting step of KdpFABC, the E1-P/E2-P transition. More recently, combining cryo-EM with biochemical and MD simulation data, allowed us to elucidate in more depth how both subunits are coupled and how they are regulated by an off-cycle state, strengthening the idea that KdpB is an early descendant of a common ancestor of cation pumps.This work was done in collaboration with the group of Inga Hänelt at the Goethe University in Frankfurt, Germany.
see:
Silberberg et al., eLife (2022)
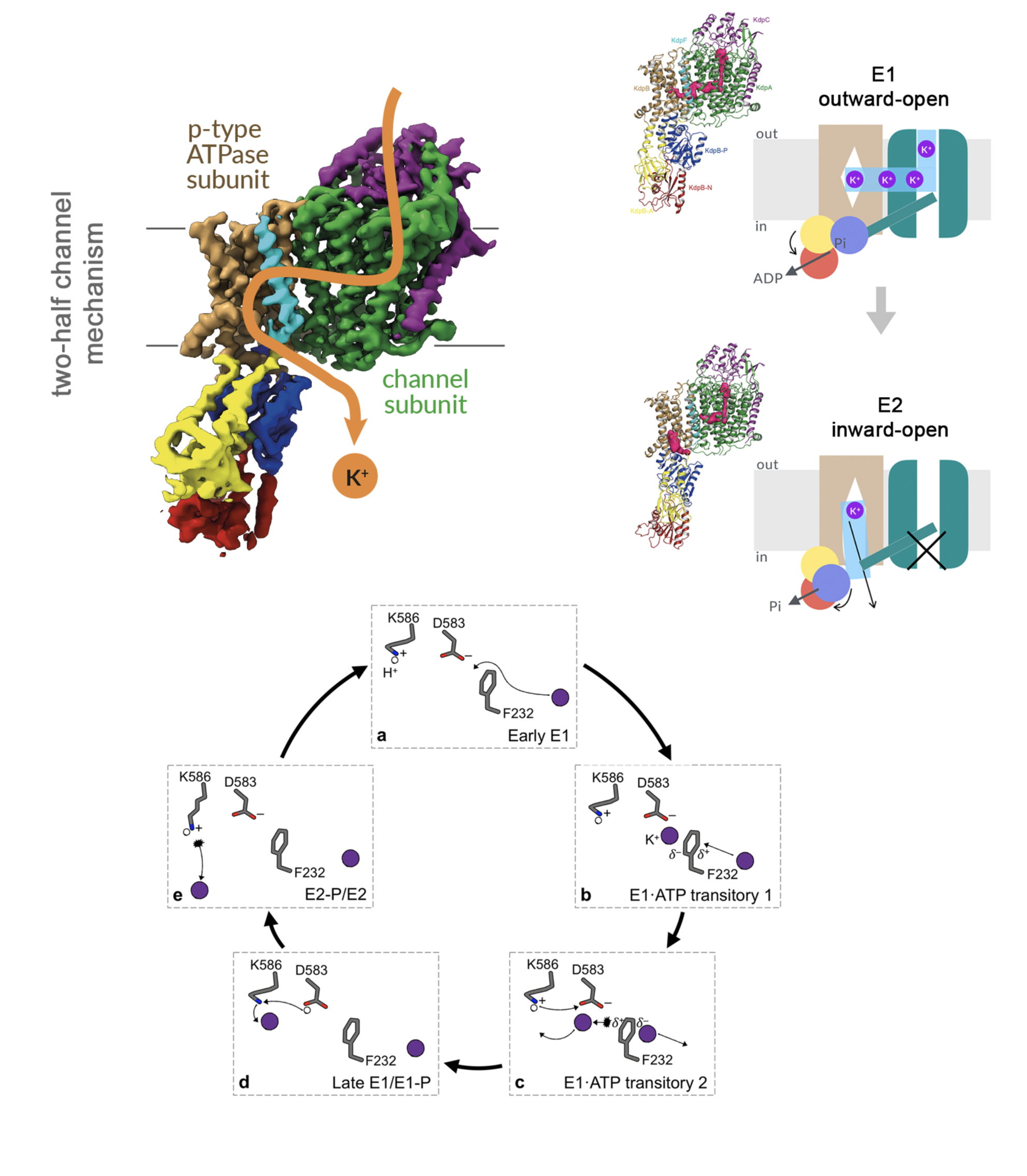
__________

TMEM16
Groovy Channels & Scramblases
The eukaryotic TMEM16 family is capable of a remarkable functional dichotomy, where members can work as Ca2+-activated Cl–-channels or both as Ca2+-activated Cl–-channels and lipid scramblases. The latter catalyse the bidirectional diffusion of lipids, i.e. phosphatidylserine, between both membrane leaflets. Our structures accompanied by comprehensive biochemical, functional and electrophysiology data, provided a great step forward in understanding the mechanism of action and regulation of TMEM16 proteins. Structures obtained at activating and non-activating conditions allowed us to identify regions that are directly linked to ligand-binding, and which regulate anion conduction via an electrostatic barrier in TMEM16 ion channels. Further, by contrasting our data obtained for TMEM16 channel to those of TMEM16 scramblases/channels, we were able to unravel how a similar protein architecture can adapt to exert these different tasks. We demonstrate how in some family branches both functions are likely mediated by distinct conformations, which we have termed the alternating pore/cavity mechanism. Whereas in the scramblase state we observe a membrane-spanning and membrane-accessible cavity through which lipids can slide, the furrow is closed in the Cl–-channel structure to form a pore that allows the diffusion of ions through the membrane. By exploiting the advantages of cryo-EM we obtained insights into the dynamics present during lipid translocation and how they might be regulated, ultimately allowing us to propose a stepwise activation mechanism. Here, we demonstrate how the protein interacts with lipids and distorts the membrane, thereby decreasing the energy barrier for lipid movement. Finally, we postulate that in other branches of the TMEM16 family both functions might be mediated by the same protein conformation.This work is done in collaboration with the group of Raimund Dutzler at the University of Zurich, Switzerland.
Kalienkova V et al; JMB 2021 (review)
__________
cryo-EM
Sample thickness measurements for optimized data acquisition workflow
While it is known that sample thickness is a key player for cryo-EM data quality, this parameter is not taken into account during common data collections. We have set up an optimized data collection workflow for single particle cryo-electron microscopy (cryo-EM), which maximizes data collection efficiency. Via our approach, sample thickness can be determined before data acquisition, and this information used to identify optimal regions and restrict data collection to images with preserved high-resolution details. This quality over quantity approach, almost entirely eliminates the time- and storage-consuming collection of suboptimal images, which would be discarded at a later stage. This strategy is especially useful, if the speed of data collection is restricted by the microscope hardware and software, or if data transfer, data storage and computational power are a bottleneck. We were able to successfully implement it in commonly used data acquisition packages and make it compatible with the majority of commercially available electron microscopes via the open-source software SerialEM. Since several years, we have used this workflow in all projects derived from our group proving its versatility and efficiency experimentally.
see:
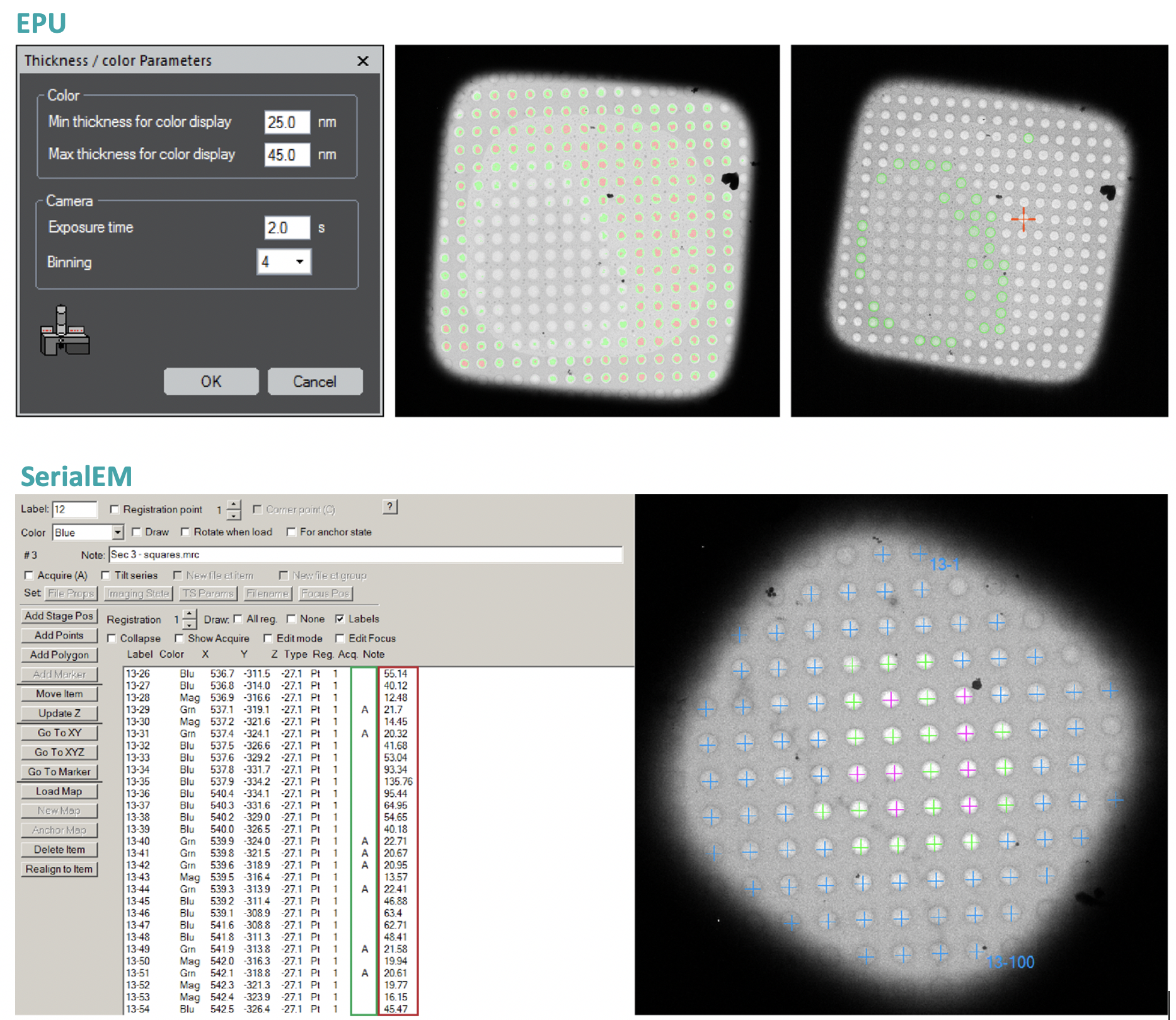

Cryo-EM studies of membrane proteins at 200 keV
Single-particle cryogenic electron-microscopy (cryo-EM) has emerged as a powerful technique for the structural characterisation of membrane proteins, especially for targets previously thought to be intractable. Taking advantage of the latest hard- and software developments, high-resolution three-dimensional (3D) reconstructions of membrane proteins by cryo-EM has become routine, with 300-kV transmission electron microscopes (TEMs) being the current standard. The use of 200-kV cryo-TEMs is gaining increasingly prominence, showing the capabilities of reaching better than 2 Å resolution for soluble proteins and better than 3 Å resolution for membrane proteins. Here, we highlight the challenges working with membrane proteins and the impact of cryo-EM, and review the technical and practical benefits, achievements and limitations of imaging at lower electron acceleration voltages.
see:
Thangaratnarajah et al., Curr Opin Struc Biol 76, 102440 (2022)
__________
Coming soon
There are so many more things to explore in transporters and channels. So much still to understand. Stay tuned to see on what else we are working…
PUBLICATIONS
Check out the Paulino Lab on Google Scholar here
‡corresponding author *co-first author
Publication
Citation Badge Altmetric Badge
Kalienkova, V., Peter, M. F., Rheinberger, J. & Paulino, C‡; Structures of a sperm-specific solute carrier gated by voltage and cAMP. Nature 623, 202–209 (2023); DOI:10.1038/s41586-023-06629-w
Thangaratnarajah C, Nijland M, Borges-Araújo L, Jeucken A, Rheinberger J, Marrink SJ, Souza PCT, Paulino C‡ & Slotboom DJ‡; Expulsion mechanism of the substrate-translocating subunit in ECF transporters. Nat Comms, 4484 (2023); DOI:10.1038/s41467-023-40266-1
Arndt, M, Alvadia C, Staub M, Clerico Mosina V, Paulino C, Dutzler R‡; Structural basis for the activation of the lipid scramblase TMEM16F. Nat Comms, 6692 (2022); DOI:10.1038/s41467-022-34497-x
Silberberg JM, Stock C, Hielkema L, Corey RA, Rheinberger J, Wunnicke D, Dubach VRA, Stansfeld PJ, Hänelt I‡, Paulino C‡; Inhibited KdpFABC transitions into an E1 off-cycle state. eLife, 6692 (2022); DOI:10.7554/eLife.80988
Thangaratnarajah, C.‡, Rheinberger, J. & Paulino C‡; Cryo-EM studies of membrane proteins at 200 keV. Curr Opin Struc Biol 76, 102440 (2022); DOI:10.1016/j.sbi.2022.102440
Walter JD*, Hutter CAJ*, Garaeva AA*, Scherer M, Zimmermann I, Wyss M, Rheinberger J, Earp J, Egloff P, Sorgenfrei M, Hürlimann LM, Gonda I, Meier G, Remm S, Thavarasah S, Zimmer G, Slotboom DJ, Paulino C‡, Plattet P‡, Seeger MA‡; Biparatopic sybody constructs neutralize SARS-CoV-2 variants of concern and mitigate emergence of drug resistance. EMBO Reports (2022) e54199; DOI:10.15252/embr.202154199
Garibsingh RAA, Ndaru E, Garaeva AA, Shi Y, Zielewicz L, Zakrepine P, Bonomi M, Slotboom DJ, Paulino C‡, Grewer C‡, Schlessinger A‡, Rational design of ASCT2 inhibitors using an integrated experimental-computational approach, PNAS 118(37) (2021); DOI:10.1073/pnas.2104093118
Thangaratnarajah C, Rheinberger J, Paulino C‡, Slotboom DJ‡, Insights into the bilayer-mediated toppling mechanism of a folate-specific ECF transporter by cryo-EM, PNAS 24, 118(34) (2021) DOI:10.1073/pnas.2105014118
Silberberg JM*, Corey RA*, Hielkema L*, Stock C, Stansfeld PJ‡, Paulino C‡ and Haenelt I‡, Deciphering ion transport and ATPase coupling in the intersubunit tunnel of KdpFABC. Nat Commun 12, 5098 (2021) DOI:10.1038/s41467-021-25242-x
Rheinberger J, Oostergetel GT, Resch GP, Paulino C‡; Optimized cryo-EM data acquisition workflow by sample thickness determination. Acta Cryst. D 77, 565-571 (2021) DOI:10.1107/S205979832100334X
Kalienkova V‡, Clerico Mosina V, Paulino C‡; The groovy TMEM16 family: molecular mechanisms of lipid scrambling and ion conduction. Review J Mol Biol: 166941 (2021) DOI:10.1016/j.jmb.2021.166941
Lam AKM‡, Rheinberger J, Paulino C‡, Dutzler R‡, Gating the pore of the calcium-activated chloride channel TMEM16A; Nat Comms 12: 719–13 (2021) DOI:10.1038/s41467-020-20787-9
Hielkema L, Heuser T, Paulino C, Walter A, Cryo-Transmission Electron Microscopy. Book chapter: in IoP-IPEM ebook series in Physics and Engineering in Medicine and Biology. Imaging Modalities for Biological and Preclinical Research: A Compendium Volume 1, Pages I.5.b-1 to I.5.b-17 (2021) DOI 10.1088/978-0-7503-3059-6
Sikkema H, van den Noort M, Rheinberger J, Paulino C‡, Poolman B‡, Gating by ionic strength and safety check by cyclic-di-AMP in the ABC transporter OpuA. Science Advances 6, 47 eabd7697 (2020) DOI:10.1126/sciadv.abd7697; press release
Kalienkova V., Alvadia C., Clerico Mosina V., Paulino C. Single-Particle Cryo-EM of Membrane Proteins in Lipid Nanodiscs. Book Chapter in Expression, Purification, and Structural Biology of Membrane Proteins. Methods in Molecular Biology, vol 2127, (2020). Springer, Nature DOI:10.1007/978-1-0716-0373-4_17
Garaeva AA, Guskov A, Slotboom DJ‡ and Paulino C‡ A one-gate elevator mechanism for the human neutral amino acid transporter ASCT2. Nature Comm 10, 3427 (2019) DOI:10.1038/s41467-019-11363-x; press release
Alvadia C*, Lim NK*, Clerico Mosina V*, Oostergetel GT, Dutzler R‡ and Paulino C‡, Cryo-EM structures and functional characterization of the lipid scramblase TMEM16F. eLife 8, 213 (2019) DOI:10.7554/eLife.44365; press release
Kalienkova V, Clerico Mosina V, Bryner L, Oostergetel GT, Dutzler R‡ and Paulino C‡, Stepwise activation mechanism of the scramblase nhTMEM16 revealed by cryo-EM. eLife 8, 213 (2019) DOI:10.7554/eLife.44364; press release
Stock C*, Hielkema L*, Tascon I*, Wunnicke D, Oostergetel GT, Askargorta M, Paulino C‡ and Haenelt I‡, Cryo-EM structures of KdpFABC reveal K+ transport mechanism via two inter-subunit half-channels. Nat Comm 9, 4971 (2018) DOI:10.1038/s41467-018-07319-2; F1000 Prime Recommendation; press releases
Garaeva AA, Oostergetel GT, Gati C, Guskov A‡, Paulino C‡ and Slotboom DJ‡, Cryo-EM structure of the human neutral amino acid transporter ASCT2. Nat. Struc. Mol. Biol. 25, 515-521 (2018) DOI:10.1038/s41594-018-0076-y; press release
Deneka D*, Sawicka M*, Lam AKM, Paulino C and and Dutzler R, Structure of a volume-regulated anion channel of the LRRC8 family. Nature 558, 254-259 (2018) DOI:10.1038/s41586-018-0134-y; press release
Paulino C, Kalienkova V, Lam AKM, Neldner Y and Dutzler R, Activation mechanism of the chloride channel TMEM16A revealed by cryo-EM. Nature 552, 421-425 (2017) DOI:10.1038/nature24652; press release
Paulino C, Neldner Y, Lam AKM, Kalienkova V, Brunner JD, Schenck S and Dutzler R, Structural basis for anion conduction in the calcium-activated chloride channel TMEM16A. eLife 6 e26232 (2017) DOI: 10.7554/eLife.26232; Selected for an eLife Insights
Paulino C*, Wöhlert D*, Kapotova E, Yildiz Ö and Kühlbrandt W, Structure and transport mechanism of the archaeal Na+/H+ antiporter MjNhaP1. eLife 3 e03583 (2014) DOI:10.7554/eLife.03583
Paulino C and Kühlbrandt W, pH and sodium-induced changes in a sodium/proton antiporter. eLife 3, e01412 (2014) DOI:10.7554/eLife.01412
Calinescu O, Paulino C, Kühlbrandt W, Fendler K, Keeping it simple – transport mechanism and pH regulation in Na+/H+ exchangers. J Biol Chem 289, 13168-14176 (2014) DOI:10.1074/jbc.M113.542993
Goswami P*, Paulino C*, Hizlan D, Vonck J, Yildiz Ö, Kühlbrandt W, Structure of the archaeal Na+/H+ antiporter NhaP1 and functional role of transmembrane helix 1. EMBO J 30, 439-449 (2011) DOI:10.1038/emboj.2010.321